
Editor: Saarelainen Lauri
Authors:
- Autioniemi Juha, BSc, Project engineer (ICT), Smart Built Environment-expertise group, School of Arctic Natural Resources, Lapland University of Applied Sciences
- Autioniemi Matti, BSc, Co-Founder, Chief Executive Officer, CTO, Aurora Powertrains Ltd, Rovaniemi, Finland
- Saarelainen Lauri, MSc, Senior Lecturer, Project manager, Civil engineering, Smart Built Environment-expertise group, School of Arctic Natural Resources, Lapland University of Applied Sciences
Type: Compilation
Publisher: Lapin ammattikorkeakoulu Oy / Lapland University of Applied Sciences Ltd
Year of publication: 2023
Serie: Pohjoisen tekijät – Lapin ammattikorkeakoulun julkaisuja 16/2023 / The Northern Factors – Publications of Lapland University of Applied Scences 16/2023
ISBN: 978-952-316-479-6 (pdf)
ISSN: 2954-1654 (on-line publication)
PDF-link: http://www.lapinamk.fi/loader.aspx?id=b9125671-e759-4ccd-8a4d-053c664d08e9
Rights: CC BY 4.0
Language: English
Summary
This publication describes in four articles the work done in Smart Charge project in Lapland University of Applied Sciences, Rovaniemi campus, and Arctic University of Norway, Narvik campus.
The Smart Charge project is presented describing the actions performed and publications prepared. The vehicle to grid (V2G) -charger has been the thread of the project and vehicle manufacturers point of view is presented when development work of theV2G-control unit is presented. Also the setup and instrumentation of the pilot test site is described in the third article. Finally a brief overview of battery development phases of portable devices and electric vehicles is presented.
Contents
Lauri Saarelainen
Smart Charge -project
Matti Autioniemi
Development of V2G-control unit
Juha Autioniemi
Lehtojärvi pilot site
Lauri Saarelainen
Battery technology overview
Initially
The demand to restrain greenhouse gas (especially CO2) emissions and global warming has raised into the focus deduction of fossil fuel consumption both in energy production and as passenger vehicle moving power. Governments are boosting this green transformation with acquisition subventions to citizens investing in electrically driven vehicles. Vehicle manufacturers are launching new models of electric vehicles (EVs) and other means of transport with an accelerating cycle. Toyota Prius in 1996 was the first step: a small capacity battery was combined with combustion engine to provide hybrid electric vehicle (HEV) without possibility to charge batteries externally. Latest development has led to plugin hybrids (PHEVs) and battery electric vehicles (BEVs) with possibility to charge the batteries from electric network. Each step has enlarged the capacity of battery pack enclosed and extended the range of travel with electric power. The popularity of EVs is growing rapidly, but it takes decades to convert the existing fleet of vehicles to non-fossil fuel driven. Hydrogen is supposed to be next step forward, but still remains “ten years ahead” as the depletion of crude oil has anticipated to happen from the beginning of 1970´s.
Charging of EVs has also raised concerns about capacity and performance of electrical network when numerous EVs are charged from the grid. Several studies have predicted that the capacity and reliability of network will suffer if EV charging is done uncontrollably, which means that charging is done randomly according to users´ demands and without connection or control with the electric grid. At the same time is expected that the lifespan of electrical grid components can suffer from repeating overloads.
Vehicle to grid (V2G) technology changes the one directional charging to two directional by adding the possibility to move energy not only from the network to battery but also from battery to network, if necessary. If controlled comprehensively this capability will compensate for the disadvantages that the exploding number of EVs will cause to electrical network. EVs can be charged from the network during low demand (usually during night-time) and provide power to network in peak load hours. Batteries connected to the grid offer a fleet of well distributed local energy storages that can compensate peak demand locally and simultaneously stabilize network behavior. This demands smart control technology and exchange of information over V2G charger.
The purpose of this publication is to summarize recent development in the area of battery technologies and Vehicle-to-Grid (V2G) -battery chargers among related industries. Publication is related to Smart Charge -project, which is presented in more detail in first chapter of this publication. In addition a brief review into the existing battery technologies is presented.
V2G-technology is presented by Matti Autioniemi from Aurora Powertrains Ltd., which is a manufacturer of electrically driven snowmobiles in Rovaniemi, Finland. In this article the technology is described from point of view of a vehicle manufacturer.
Smart Charge project has built a demonstration environment to Arctic Snow Hotel in Lehtojärvi. Charging system arrangement together with the data visualization development is described in a chapter written by Juha Autioniemi from Lapland University of Applied Sciences, Rovaniemi Campus.
The Arctic University of Trondheim (UiT) has been in responsible to analyze collected data from pilot sites in Longyearbyen and Lehtojärvi. List of their articles and activities is enclosed in the first chapter describing Smart Charge project. There will be an article to be published later on concerning Laehtojärvi case during spring 2023.
Lauri Saarelainen
project manager of Smart Charge project
Lauri Saarelainen
Smart Charge project
This chapter describes overall features of Smart Charge project, which was carried out to create new information about Vehicle-to-Grid (V2G) -solutions commercial and technical possibilities and challenges with the help of three pilot studies located in Finland, Sweden and Norway. In pilot sites intelligent local systems were actualized to use the electrical vehicles (snowmobiles) both as an program service (snowmobile safaris for tourists) and an energy storage (to improve local electric grid flexibility and quality of energy) while simultaneously evaluating the commercial and technical aspect of the system accomplished.
Leading party in this project was Lapland University of Applied Sciences (Lapland UAS) and Norwegian partner was The Arctic University of Norway in Tromsø (UiT). Smart Charge -project was funded by Interreg V A Nord 2014-2020 program, Research and Innovation field of operation and national funding to Lapland UAS came from Regional council of Lapland, Regional European Development Fund ERDF.
– Funding to Lapland UAS was admitted 278.745 € (100%) and divided between Interreg Nord 181.190 € (65%), Regional Council of Lapland 55.751 € (20%) and Lapland University of Applied Sciences 41.813 € (15%).
– Funding to UiT was 234.390 € (1.930.000 NOK) divided fifty-fifty between Interreg Nord 117.195 € (965.00 NOK) and UiT 117.195 € (965.000 NOK)
– Total funding for the Smart Charge -project was 513.144 € (both parties included).
Total expenditure of funds realized as Lapland UAS total was 237.140 € (-15% from budgeted) and UiT total was 236.483€ (+0,8% from budgeted). The two most important reasons for this were that originally budgeted Jukkasjärvi pilot test site in Sweden was cancelled and prevailing covid-19 pandemic restrictions made travels almost impossible to actualize.
Project was carried out between 1st of February 2019 and 31st of July 2022. Original date of termination was 31st of January 2022, but extension period of six months was applied for and accepted by the providers of funds 20th of October 2020.
General scope of the Smart Charge project was to promote intelligent and energy efficient solutions implementation when responsible services are provided within construction, energy, vehicle and tourism sectors. Main goal was to provide new information about Vehicle-to-Grid (V2G) -solutions commercial and technical possibilities and challenges with the help of three pilot studies. Due to technical and resource reasons only two pilot sites were finally implemented: one in Svalbard Norge and other in Lehtojärvi Finland. More detailed description of Lehtojärvi pilot site is enclosed in later pages of this publication.
In these pilot sites intelligent local data collection and power distribution systems were constructed and information was collected, analyzed and visualized to provide and promote private enterpreneurs investements towards renewable energy efficient solutions, which would also decrease CO2-emissions as well. Simultaneously optimum V2G-concept was designed and tested, which is based on open concept and protocol standards. More detailed description is enclosed in later chapter of this publication.
Accomplished results were distributed and presented in various seminars, webinars and conferences and publications both in Norway and Finland and also nationwide. List of publications and public presentations where Smart Charge was presented is as follows.
-Smart Charge project kick-off seminar held in Levi, Kittilä 4.-6.4.2019 with interest groups involved
-Three project workers participated the Battery Show / Electric & Hybrid Vehicle Technology Europe 2019 -fair in Stuttgart 6-9.5.2019
-“E-mobility in the Arctic” Smart Charge webinar, focusing on e-mobility in the Arctic region was held on 21-22 October 2020 (in English) where 60 persons were participators from 9 European countries https://www.tequ.fi/en/project-bank/smartcharge/webinar/
– Smart Charge Webinar – UiT, Campus Narvik with NCE (Norwegian Center of Expertise) Smart Energy Markets was held on 11 March 2021 (in Norwegian) was aimed to the Norwegian energy industry and over 70 persons were attending.
-“TEQU Talk – eLapland 2030 – Electronic transport is becoming more common – how Lapland municipalities should prepare” webinar was held on 25 March 2021 (in Finnish) where 90 persons were online audience https://www.tequ.fi/fi/tequ-talk-elapland-2030/
State-of-the-art presentations about e-mobility, batteries and energy flexibility (Bernt A. Bremdal)
-For Troms & Finnmark fylkeskommune (June 2021)
-For the Norwegian Association of Public transport (June 2021)
-For Ruter – the public transport service of Oslo (August 2021)
Presentation about Emission free mobility: Climate friendly, user and system friendly (Bremdal)
-For Vågan kommune – Lofoten (October 2021)
– Shayan Dadman, Bernt A. Bremdal, Kristoffer Tangrand. 2012 “The role of electric snowmobiles and rooftop energy production in the Arctic” presented at SEGE2021 & CGEE2021 The combined international Conference on Smart Energy Grid Engineering and Conference of Clean and Green Energy Engineering, August 11-13, 2021. http://www.cgee.org/cgee2021.html
– 4th International Conference on Smart Energy Systems and Technologies (SEST): webinar held 6-8 September 2021, Vaasa, Finland (project manager participation in audience)
– Arja Kotkansalo, Juha Autioniemi, Tuomas Sinisalo. 2021.“Smart Charge-Intelligent charging”. Lapland University of Applied Sciences publication series B vol. 11/21 “eLapland 2030 – webinar summary” (in Finnish) p. 37-45. ISBN 978-952-316-401-7 https://www.lapinamk.fi/fi/Yrityksille-ja-yhteisoille/Julkaisut/Lapin-AMKin-julkaisut/Tekniikka-ja-teollisuus?itemid=2669&showlocation=35503cbb-50cd-4dcf-8fa1-c4e2933a282b
— Shayan Dadman, Bernt A. Bremdal, Kristoffer Tangrand. 2012 “The role of electric snowmobiles and rooftop energy production in the Arctic”. Journal of Clean Energy Technologies. JOCET 2021 Vol.9(4): 46-53 ISSN: 1793-821X http://www.jocet.org/index.php?m=content&c=index&a=show&catid=83&id=897
– B. A. Bremdal, I. Iliana, K. Tangard and S. Dadman: E-Mobility and Batteries – A Bussiness Case for Flexibility in the Arctic Region. World Electric Vehicle Journal, Feb. 2023 https://www.mdpi.com/2032-6653/14/3/61
– B. A. Bremdal, S. Dadman: Using Light Electric Vehicles for V2G services in the Arctic. Submitted to the CIRED 2023 Conference.
– S. Dadman: Using Deep Learning to Forecast Solar Energy Production in The Arctic, Technical Note, The Arctic University of Norway, 10.12.21. (Basis for a paper, yet to be published.)
– F. Safarpour, B.A.Bremdal Smart Charge State-of-the-Art Report, Third edition, July 2022. Replaces the one from May 2021
Matti Autioniemi
Development of V2G-control unit
Vehicle to grid or V2G means bidirectional power transfer (BPT) between an electric vehicle (EV) battery and the power grid. There are also other similar applications shown in Figure 1 including vehicle-to-load, vehicle-to-home, vehicle-to-building and vehicle-to-vehicle depending on where the EV battery is connected to. Sometimes term vehicle-to-x (V2X) is used instead of V2G or one of the other use cases.

Figure 1. Overview of different charging scenarios.
(https://535485.smushcdn.com/2232832/wp-content/uploads/2021/02/ANUrevsReportchartgraphic-640×491.jpg?lossy=1&strip=0&webp=1, 24.11.2022)
Depending on the components onboard the electric vehicle, the power can be transferred bidirectionally through the AC or DC bus of the vehicle charging inlet. Bidirectional AC requires integration of DC/AC conversion into the EV onboard charger (OBC) to enable power transfer from battery towards grid. There’s not many such OBCs on the market yet and the variety of national grid codes makes the implementation more complicated. On the other hand, bidirectional DC charging is more straightforward and there are some existing solutions already today. CHAdeMo standard has been the leader so far in V2G and there are some V2G compatible vehicles and stations. However, the CHAdeMo is losing ground to CCS standard which is becoming the dominant standard in EU and North America and CCS inlet can be found from basically all new electric vehicles and charging stations. Figure 1 shows the variety of existing standard AC and DC sockets in different regions. Despite the different physical dimension of the CCS inlet in USA and EU, the communication protocol remains the same so manufacturers just need to adopt the correct inlet type for the geographical region.
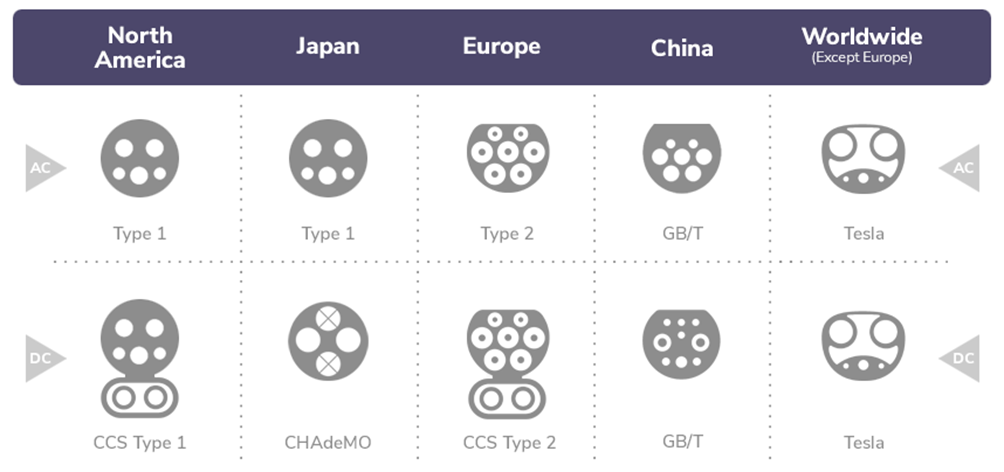
Figure 2. Overview of different AC and DC inlets used around the world. (https://www.joosup.com/wp-content/uploads/2022/08/EV-Charger-Socket-Chart.png, 24.11.2022)
CCS stations typically use DIN 70121 communication protocol which has a limited set of features compared to the recently updated ISO 15118 standard. They both communicate using powerline communication (PLC), so most of the existing CCS compatible chargers/vehicles already have ISO 15118 compliant hardware and require just software updates.
In April 2022, a major addition to ISO15118 standard was published with the release of ISO15118-20 describing the second generation V2G communication interface and especially Plug&Charge and BPT features. The Plug&Charge feature will bring smoother charging experience as the identification process doesn’t necessarily need additional apps, cards, or tags anymore. The EV will carry the identification details and will communicate them to the Electric Vehicle Supply Equipment (EVSE) when plugged in, so no user interaction is required. The same identification or certificate can be used for multiple contracts making the need for multiple different tags/apps obsolete. The new standard brings also enhanced data security by means of mandatory TLS 1.3 compared to minimum TLS 1.2 earlier. The security keys/certificates are also more secure now. Other new features include support for wireless power transfer (WPT), automated connection device (ACD) and dynamic mode. In the dynamic mode shown in Figure 3, the EV gives control of the ongoing session to the charging station. The station can then control the power setpoints with minimum delay which is very useful for smart V2G-services. The BPT feature describes the communication protocol and process to transfer power bidirectionally between EV and EVSE. This feature enables the V2G-applications described earlier in the article in a fully standardized way compared to manufacturer specific solutions with the previous versions of DIN 70121 and ISO 15118 standards.
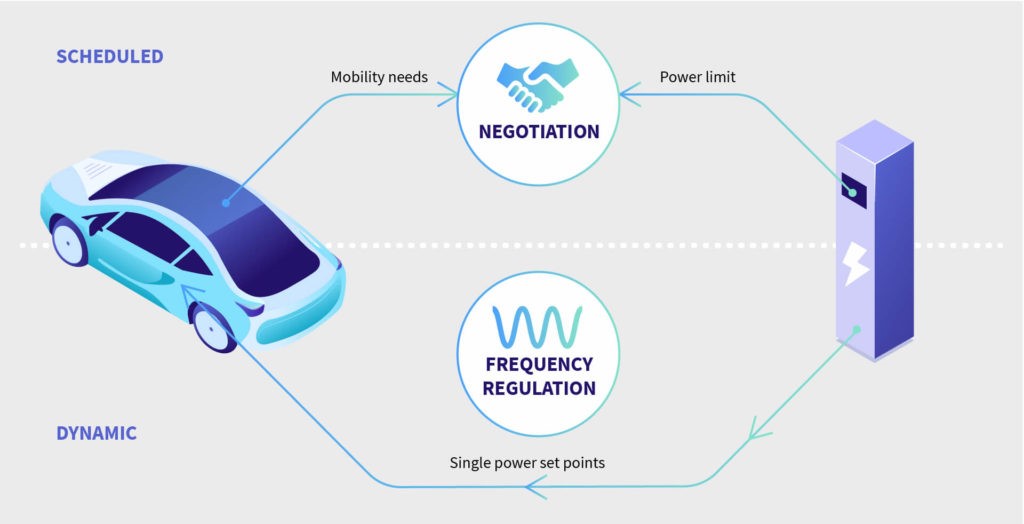
Figure 3. ISO15118-20 has scheduled and dynamic charging modes.
(https://www.switch-ev.com/blog/new-features-and-timeline-for-iso15118-20, 24.11.2022)
There’s also interesting and yet not widely used feature described in the IEC61851-1 Annex D called LIN-CP or Local Interconnect Network on the Control Pilot. As the name implies, it is a digital LIN bus over the Control Pilot (CP) line and is basically a low-cost upgrade for the PWM control signaling. It is backwards compatible with the PWM and maintains the same CP voltage levels as described in the IEC61851-1 Annex A. LIN-CP is also used in the North American SAE J3068 standard but there are some differences which are described in J3068 Appendix F. In any case, this digital communication protocol would be useful enabling smarter AC/DC charging at low cost as you can transfer relevant parameters digitally over the LIN bus. One additional use case for the LIN-CP would be to use it in combination with ISO15118-20 to speed up the Plug&Charge authentication process by initiating the authentication over LIN-CP to quickly identify the EV connected to the respective EVSE. Unfortunately, there’s not too many electric vehicles equipped with LIN-CP hardware and supporting the protocol.
Aurora Powertrains, the developer and manufacturer of eSled – electric snowmobiles and battery modules, has developed Vehicle Charge Control Unit (VCCU) displayed in Figure 1 to handle charging process of the eSled. VCCU is a credit card sized component integrated into the eSled and it handles the communication between the eSled Vehicle Control Unit (VCU) and EVSE. On the vehicle side, it communicates using CAN-bus, and on EVSE side it communicates using PLC. It is compliant with the ISO15118 including the latest update and is also compatible with the DIN 70121 standard. VCCU is also compliant with the IEC61851 including the LIN-CP communication described in the Annex D, and also with the SAE J3068.

Figure 4. VCCU – Vehicle Charge Control Unit. Rendering of a finished product on the left and a prototype in test bench on the right.
The VCCU has been integrated into eSled and has been used in the Smart Charge project for real-life testing in eSled safari operations at Lehtojärvi pilot site near Rovaniemi in Finland. The pilot site has one Venema V2G CCS charger installed during the project and it has been one of the main charging stations to test and develop the VCCU operation and bidirectional charging. Project has also acquired one V2G AC station with LIN-CP communication protocol, and it has been used in the development and testing of the LIN-CP protocol software of VCCU in laboratory conditions. Aurora has also collaborated with Vattenfall R&D team in the project to comply with the latest standards and discuss V2G use cases which should be tested and demonstrated at the pilot site. These integrations and project results has enabled Aurora Powertrains to apply and receive an ECSEL JU grant for Energy ECS project together with other partners to further develop VCCU and to demonstrate smart V2G-charging in fleet scale electric snowmobile safari operations. Aurora Powertrains will integrate the VCCU to its new eSled model shown in Figure 2 and is planning to launch it as a separate product in 2023.

Figure 5. Front view of the new eSled – electric snowmobile model
Juha Autioniemi
Lehtojärvi pilot site
Overview
Lehtojärvi pilot site is developed in Smartcharge project as a place to test V2G chargers, test and develop V2G charging in both directions, collect detailed data of the process and develop and test smart-grid functionality. Pilot site is located at Aurora Powertrains premises in Lehtojärvi, Finland. Pilot site is connected to main power grid via 200 A electricity contract. Locally power is distributed from outdoor electric cabinet mainly via five 63A three phase sockets and one 63 A connection to safari house on-premises. Pilot site enables testing and developing V2G charging solutions using either Venema V2G charging station (DC) or Nuvve PowerPort (AC). Main focus is in DC and CCS based V2G charging.
Data is collected from both energy consumption and production. Monitored consumers are electric snowmobiles that are connected to the chargers and safari house. Production data is gathered from a local reference solar power plant and from a V2G-charging station. A simplified system architecture is presented in figure 1. All data collected at the site is forwarded to Lapland UAS cloud platform via gateway, that supports wireless connectivity.
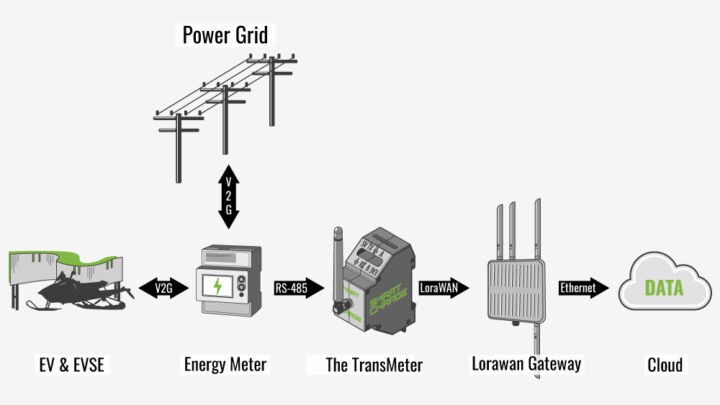
Figure 1. Lehtojärvi system architecture.
Pilot site data acquisition
Ursalink UG87 LoRaWAN gateway (Figure 2). was chosen as a central communication point at pilot site. Gateway is robust outdoor unit to withstand harsh environments. It supports creating a local private LoRaWAN network that can easily cover quite a large area. Public LoRaWAN network is also available in the area, but it is not suitable for real-time data acquisition that is required at pilot site due to regulations and limits that come with public LoRaWAN network. Gateway has 4G connectivity for data forwarding to cloud back-ends.
The main energy and power measurement devices are ABB two-way meters. Data from meters is collected with a TransMeter device (figure 2) developed in Lapland UAS laboratory during the SmartCharge project. TransMeter is based on a Pycom LoPy v4 IoT-board with added RS485 communication and enclosure and connectors for typical DIN-rail installation in electric cabinet. Single TransMeter can collect data from a single or multiple energy meters.
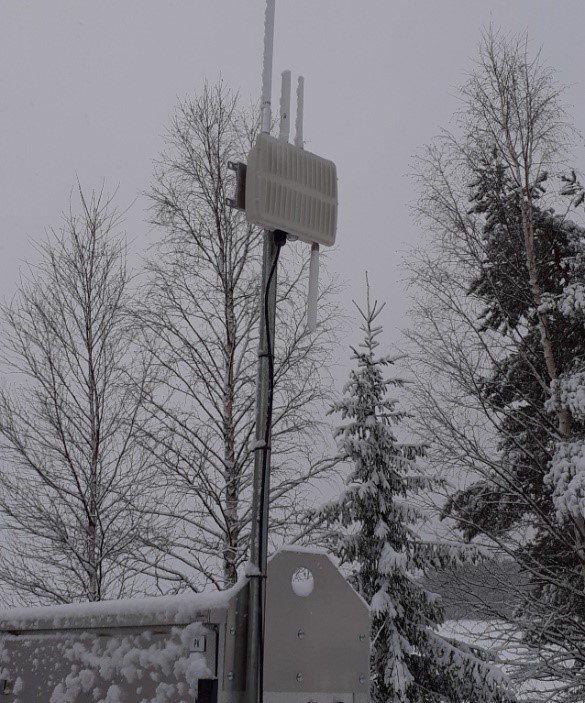
Figure 2. 4G-gateway device

Figure 3. TransMeter device
Local reference solar panel system consists of two Fronius Symo solar inverters each connected to 20 kWp solar panel array. Inverters are connected to Fronius Solarweb via separate 4G mobile router and are configured to push data directly to Lapland UAS cloud platform API. Solar panels are facing east and produces energy from April to November based on data presented in Figure 3 from year 2021. Total energy production at year 2021 was 23243 kWh.
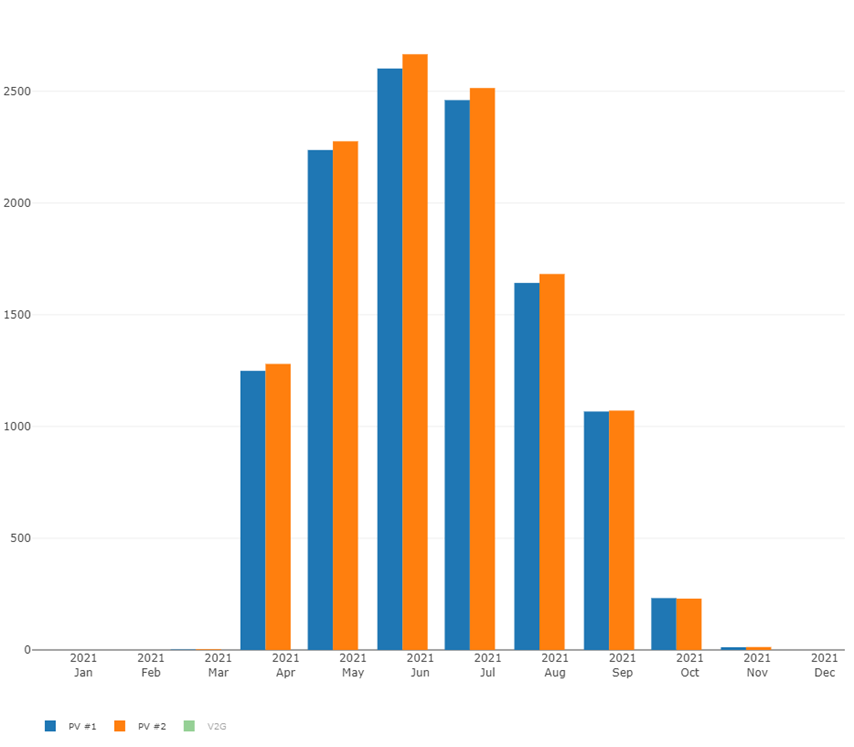
Figure 4. Lehtojärvi solar power system energy production at year 2021 [kWh].
Obviously solar energy is available from January to March too, but panels are covered with snow and it is not economically reasonable to clean them. To support electric snowmobile charging, solar system installation direction and angle should be quite different. Another option would be storing energy that has been produced at summer.
Basic environmental variables are collected at site. These are outdoor temperature and humidity and safari house temperature and humidity. Safari house has building automation system that is updated to allow remote and machine control. These updated makes it possible for building to be a part of local smart grid together with eSled´s and EV chargers.
V2G-charging station and OCPP server
Venema V2G DC CCS charger was the only available V2G charger that was possible to get during Smartcharge project due to Coronavirus and worldwide shortage of electronic components starting spring 2020. Another reason was that V2G DC CCS charging technology and standards are not consumer ready yet. We contacted several charging station suppliers during 2021 and spring 2022, and their V2G DC CCS charging stations were not available in Finland or product launch was delayed mostly due to reasons mentioned before. Contacted suppliers were ABB, Wallbox, InterControl, (Webasto and Kostal).
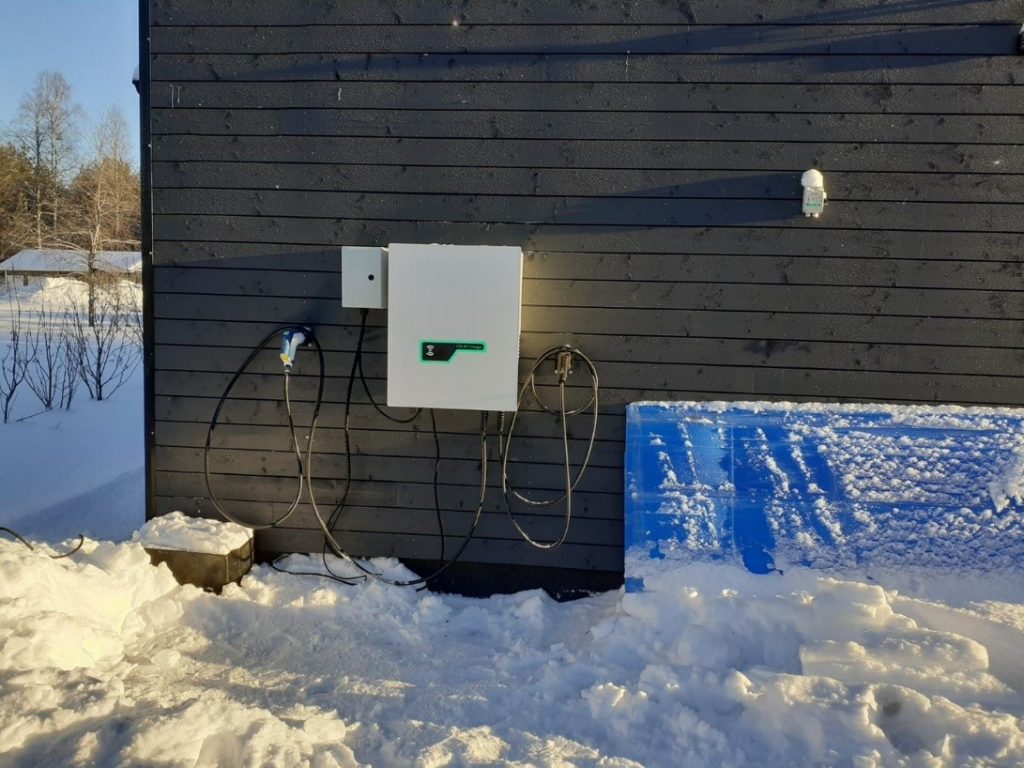
Figure 5. Venema V2G-charging station and remote connection box installed at Lehtojärvi.
Venema V2G unit at site is connected to 4G-gateway and its USB-interface is made available via remote connection for configuration and direct connection with Venema´s software. This is helpful during testing. V2G unit is connected one of the 63A sockets two-way electricity meters and also more detailed data is collected directly from V2G-charger using its Modbus interface.
During Smartcharge project OCPP v2.01 compatible OCPP server was implemented to Lapland UAS cloud environment. Venema V2G charger is connected to this OCPP server and it reports its state changes and charging events to OCPP server. Server has some basic functionality: receive transaction events, receive meter data, receive charger state events and allow remote start and stop of transactions. Server is not intended to have all OCPP functionality – its merely for testing purposes. Server is based on open-source Python implementation of the OCPP. This open source project is located in Github, https://github.com/mobilityhouse/ocpp.
Cloud platform and data visualization
All measured data from pilot site is routed to Lapland UAS cloud platform and saved to database. Cloud platform has several tools and user interfaces to interact and explore the data. Data can be exported to data analysis tools eg. DIAdem or Excel for detailed inspection.
Pilot site real time data is visualized at https://dash.tequ.fi/apps/smartcharge. Authorization is needed to access the application, please contact Smartcharge project team for access.
Smartcharge visualization application is a HTML5 web app that is running on Lapland UAS cloud platform on combined Node.js and Node-RED environment. Application front-end is served inside a Node-RED application and also back-end operations are programmed in Node-RED. Environment uses IBM Cloud App ID to authenticate users.
Application has access to all data that is collected at pilot site and application can also get data from Aurora Powertrains eSled´s that are at the site based on geofencing. Application is programmed using following libraries: Bootstrap 5 for the layout, Font Awesome 6 for the icons and graphics and plotly.js for line and bar charts. Figures 5 represents a snapshot of the application user interface, where you can see on a high level how energy flows between different producers and consumers.
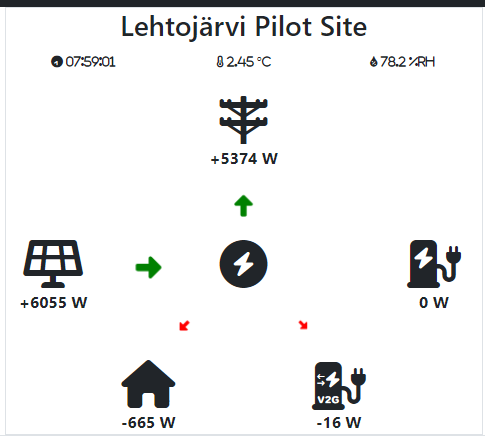
Figure 6. SmartCharge application.
Conclusion
As V2G and smart grid technologies are rapidly evolving, Lehtojärvi pilot site is a good place to develop these technologies now and also in the future. V2G and smart grids are not ready yet, there are a lot of work to do in these areas. Lehtojärvi pilot site gives its small input to this work with its special location in Lapland.
Lauri Saarelainen
Battery technology overview
The race for better EV batteries has been called the next gold rush. This chapter explains the development on battery technologies over the years. The ternd has been that battery shell voltage should be maximized while molar mass of reacting substances as well as the weight of other materials used should be as low as possible whenever mobile devices and vehicles are as an object of interest.
Following diagram 1 describes the energy intensity of different battery technologies in respect of volume and weight (mass) with cylindrical (C) and prismatic (P) type of electrode materials.
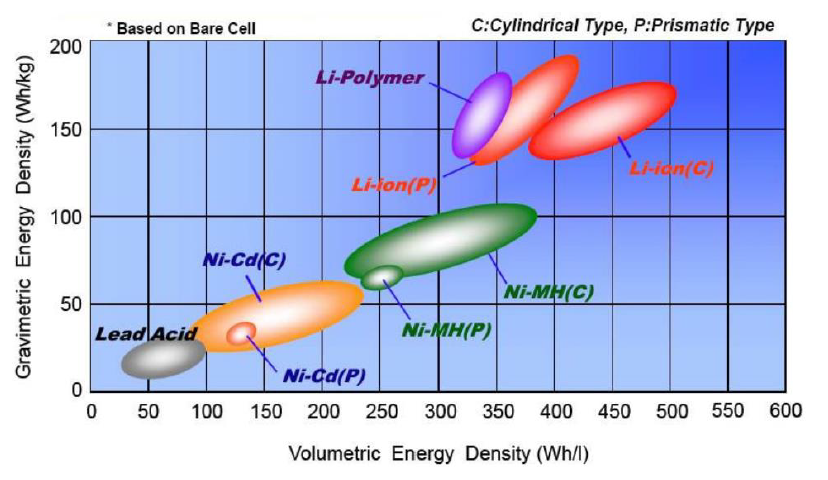
Diagram 1. Rechargeable battery technologies in respect of gravimetric and volumetric density. (Epec Engineered Technologies 2022)
Lead acid batteries
Lead acid batteries are the oldest technology but still in use because of low price and decent energy density. Battery cell electrodes are made of lead alloys and electrolyte used is mix of sulphuric acid and water. Batteries are suitable for multiple purposes where energy is needed to be emitted longer periods of time (with stand-by supply systems and solar panels) or if momentary high discharge current is needed (starting batteries of combustion engines).
When lead acid battery is fully charged, it can resist cold temperatures fairly well, but loses energy capacity remarkably in sub-zero temperatures and when discharging occurs, electrolyte dillutes and can freeze if not charged. Self-discharge is usually low, but if not used, lead sulphite will crystalize which reduces capacity and current receptivity. Capacity reduction follows from increase of internal resistance, when chemical reaction retards. (Battery university)
Lead acid batteries are developed maintenance free and with improved materials and electrode constructions and advanced manufacturing technology better corrosion and cyclic durability has been reached. Battery materials are toxic, but can be recycled and reused.
Nickel-cadmium batteries (NiCd)
Nickel-cadmium battery cell consists of a cadmium made anode and a nickel made cathode with a potassium hydroxy solution as an electrolyte. Because cadmium is toxic Ni-Cd battery use is limited to special purposes and prohibited in consumer products in EU area since 2006 by RoHS (Restriction of Hazardous Substances in Electrical and Electronic Equipment) -directive.
Ni-Cd is one of the most rugged and forgiving batteries and aviation industry is loyal to this technology. Ni-Cd batteries can tolerate 2000 load/discharge cycles, lifetime can be 8-25 years and they are used in applications, where high discharge current is needed e.g. when starting a jet turbine or large diesel engine.
Ni-Cd battery has a good resistance of charge/discharge cycles, accepts fast charging and deep discharge, it has a wide temperature range of use and tolerates long discharged storage. Disadvantages are low specific energy (cell voltage 1,2V), high self-discharge and memory effect, which lowers the output capacity if charge/discharge cycle is repeatedly done only partially. (Battery university 2022)
Nickel-metal-hydride batteries (NiMH)
Research of metal-hydrides was started late 1960s when scientists discovered that certain metal alloys can store hydrogen atoms. Instabilities with metal-hydride led to nickel-hydrogen development and new alloys discovered in 1980s improved the performance of Ni-MH which today performs at 40% higher specific energy than standard NiCd technology. (Battery university 2022.)
This does not come without drawbacks: charging is more challenging and self-discharge is among the highest compared to other battery types. If hydride materials were modified to minimize self-discharge, the specific energy was decreased. Although 1990s development was far enough to launch NiMHs onto the market for mobile devices and hybrid vehicles. NiMH has become the most common rechargeable battery type for consumer application in all shapes and sizes from the largest battery manufacturers.
NiMH battery cells are possible to connect both in series and in parallel. Battery can tolerate high pulses of current both in charging and discharging which occurs when EV or hybrid is decelerated or accelerated. Batteries are maintenance free and can tolerate overcharge and deep discharge. NiMH batteries are more environmentally friendly, have lesser memory effect than NiCd and the amount of nickel makes the recycling profitable.
Disadvantages are limited service life which gets worse with deep discharge, complex charge algorithm, poor overcharge adsorbtion but high heat generation when charged. All this means that NiMH batteries can be charged with high current up to 80% of capacity, but after that it has to be limited to prevent excess heat and gas formation.
Lithium ion batteries have replaced other techniques in portable consumer applications completely: only devices that are planned to use non-rechargeable 1,5V batteries use NiMH rechargeable batteries because of the 1,2V cell voltage which is suitable for this purpose.
Toyota is using both NiMH and Li-ion batteries in its hybrid vehicles.
Lithium ion and lithium polymer batteries
Lithium ion battery is not one uniform battery technology but a wide range of different types of technologies that differ in chemistry and materials from each other.
Lithium is in periodical table at first column so it has one electron in its outer shell which makes it very reactive metal. Lithium is the lightest metal and has the biggest electrochemical potential and energy density in respect to weight. Problem is its fragility and reactivity with other materials which made the first attempts to construct lithium-based batteries failures due to thermal control when charging. These properties delayed development for a long time, until Sony introduced the first commercial lithium ion battery in 1991 where lithium acted as an intercalation compound both at the anode and the cathode while lithium ions were moving between electrodes during charging-discharging cycles. This technical realization was safer than previous attempts to construct a lithium battery.
Suitability of carbon as an anode material was also detected: lithium ions can be joined and detached from layers of carbon atoms and the potential of this anode electrode does not differ from metallic electrodes anode voltage. The positive electrode is manufactured on the surface of a metal film with the help of carbon and powdered binding agent. Many different materials can be used as a cathode: battery types are named according to the metal alloy used in the positive electrode. Most common cathode materials are Lithium Cobalt Oxide (LiCoO2) LCO, Lithium Manganese Oxide (LiMn2O4) LMO, Lithium Manganese Cobalt Oxide (LiMnCoO4) NMC, Lithium Iron Phosphate (LiFePO4) LFP and Lithium Nickel Cobalt Aluminum Oxide (LiNiCoAlO2) NCA, Lithium Titanate (Li2TiO3)) LTO.
Cobalt provides high energy density, but low supply current. Disadvantages are high price of material, toxicity, poor tolerance of charge-discharge cycles and possibility for electrolyte to penetrate between oxide layers. Nickel on the other hand is cheaper and more recyclable than cobalt, but supply current is even lower.
Manganese is the most environmentally friendly option and because of its spinel structure it can store great amounts of lithium with good thermal stability. Manganese oxide electrode batteries can endure a stronger current than cobalt based batteries, but the capacity is smaller.
Combining the three mentioned materials (NMC-electrode) the lithium battery can be adjusted to be suitable for different purposes; compromise between high energy intensity or high load rating is always the issue. NMC is the leading cathode chemistry at the moment. (Battery University)
Electrolytes used in lithium ion and lithium polymer batteries are liquid, gel type or polymer type electrolytes. In liquid electrolytes a lithium salt is dissolved with an organic solvent. Water cannot be used because of high reactivity of lithiun and an organic electrolyte enables wider a potential range than a water-based electrolyte would.
Positive advantages of lithium ion batteries are high energy intensity and cell voltage compared to other battery technologies, high intensity in respect to weight and volume, a large temperature range, high charge-discharge cycle tolerance without losing capacity, capability to charge and discharge with high power and speed, slow self-discharge, no memory effect, closed shell structure and no need for maintenance.
Negative disadvantages relate to high reactivity of lithium: the battery exhibits impaired function in low temperatures meaning lower capacity and voltage but lower capacity occurs in high temperatures as well. Li-ion battery can represent high fire load which means that safety circuit is needed to control progression of temperatures and voltage during charging and discharging. A damaged battery can also develop thermal escape when chemicals react vigorously which damages the chemicals which can in turn lead to ignition and intense fire if battery overheats.
Lithium Polymer differs from others by the type of electrolyte used: originally in 1970s it was a solid polymer electrolyte that resembled a plastic-like film which had poor conductivity in room temperature and had to be heated up to 60oC to enable current flow. Therefore the “true plastic battery” hype didn´t become realize until a gel-type electrolyte was added. Most Li-polymer cells today consist of polymers that range from synthetic plastics to natural biopolymers and proteins that compose a microporous separator with some moisture. Li-polymer is not considered as an unique battery chemistry and can be built on many systems that are usually cobalt-based.
From user point of view, the Li-ion is same as Li-polymer; both systems use similar cathode anode materials and the same amount of electrolyte, only the microporous electrolyte replaces the porous separator. This enables a little higher specific energy and thinner construction, but manufacturing cost is higher.
Lithium nickel cobalt aluminum oxide battery NCA was developed late 1990s and offers high energy and power densities and a good life span: although high cost and marginal safety are negative characteristics, NCA is a candidate for EV powertrains and for example Tesla is using this technology.
Next diagram compares specific energies of lead-, nickel- and lithium-based systems more in detail:
– Li-aluminum NCA is the best by storing more capacity than others but only when specific energy is concerned
– Li-manganese LMO and Li-phosphate are superior in terms of specific power and thermal stability
– Li-titanate LTO has low capacity, but the chemistry beats others in terms of life span and cold temperature performance.

Figure 2: Typical specific energies of different battery types. Battery University https://batteryuniversity.com/article/bu-205-types-of-lithium-ion
Future development of batteries
Storing electrical energy economically is one of the unsolved challenges of modern society. Experimental battery technologies live only in laboratories and communicate in terms of outside world with technical papers to persuade investors or die in the lab without anyone hearing of their passing. Research and development does not only concentrate on electrode materials and chemistry but also on production methods and other manufacturing challenges as well as new type of construction possibilities of battery packs. The most promising experimental battery technologies are listed below. (Battery University 2022)
Lithium-air (Li-air)
This battery promises store remarkably more energy than Li-ion is capable by adopting fuel cell construction and extracting oxygen from air. The battery uses a catalytic air cathode, an electrolyte and a lithium anode. Similarities with other “air breathing” technologies can be found; air purity is critical and measures to ensure this demand (compressing and filtering air) reduce 30% of energy produced such as with fuel cells. Other remarkable problem is sudden death syndrome when lithium peroxide film forms a barrier for electron movement and wrecks the battery´s storage capacity. Several companies and universities are developing this technology to meet EV powertrains´ needs.
Lithium-metal (Li-metal)
High specific energy and good loading capability are unbeatable properties that set great expectations for this technology but uncontrolled lithium dendrite growth can penetrate the separator and cause safety risk of shortcuts. An electrolyte additive of nano-diamonds has been tested but better precautions are still needed to bring Li-metal batteries to consumer applications.
Solid-state Lithium
Graphite anode is limiting the specific energy and great deal of R&D is done by trying to replace it with pure lithium and substitute the liquid electrolyte soaked separator with a solid polymer or ceramic separator. Many of the problems faced here resemble those of lithium-metal batteries: poor conductivity in cold temperatures and low cycle count. Research laboratories, including Bosch, predict that solid-state could become commercially available in EV´s in 2025.
Lithium-sulfur (Li-S)
Both substances represent low or moderate atomic weight that offers three times higher specific energy than Li-ion. The anode is instead of graphite made of lithium metal and works both anode and source of lithium ions and cathode instead of metal oxide is made of sulfur. Discharging and recharging is possible in very cold temperatures and sulfur is abundantly available and environmentally friendly compared to other battery metals. Biggest problem is the low cycle count because sulfur is lost from the cathode when reacting with the lithium anode.
Sodium-ion (Na-ion)
Sodium-ion is a similar low cost li-ion alternative because of affordability and readily available sodium. Cost per kWh is comparable with lead-acid battery. However, cycle count and large volumetric expansion when fully charged need to be settled.
Lithium-manganese-iron-phosphate (LMFP)
Capacity is said to increase by 15% compared to Li-Phosphate LIFEPO4 system and low cost and good safety are other benefits to make this battery suitable for EV powertrains.
Dry Battery Electrode
Maxwell Technology (previously owned by Tesla) has developed a dry battery electrode (DBE) coating technology: dry powder is first mixed, then spread to afilm and finally the film is laminated to a current collector without any use of solvents. This process can be carried out with different electrode configurations and material loading weights and active layer thicknesses. H.Duong, J.Shin & Y.Yud 2018 http://www.powersourcesconference.com/Power%20Sources%202018%20Digest/docs/3-1.pdf
Tesla has presented its new 4680-type cylindrical cell with NCM 811 cathode chemistry (81,6% Ni, 10% both Cd and Mn, but no Al). The new dry film technology has not been used on the cathode but on the anode although it was expected to have been used on both cases (or none). Graphite is the active material anode and there is no silicon involved to boost energy density as there usually is. Munro https://www.youtube.com/watch?v=VEBY7rEUZiI This could be because of an early production sample, but still estimated energy density is between 272-296 Wh/kg and five times more than smaller 2170-type cell. Some doubts have been presented in achieving full production scale before year 2023. Insideevs.com https://insideevs.com/news/598656/tesla-4680-battery-cell-specs/
Battreries as Structural Components
Using Lithium iron phosphate as a positive electrode and carbon fiber as a negative electrode, conductor and load bearing material researchers at Chalmers University of Tehcnology have been focusing to use battery as a structural component and this way form a “massless” energy storage with future cars where bodyparts can be batteries. (Chalmers University 2022)
Carbon Nanotube Electrodes
Nawa Technologies has patented Ultra Fast Carbon electrode that uses vertically aligned carbon nanotubes that can boost battery power ten times, energy storage three times and lifecycle five times over current battery packs. Charging time is within minutes and technology could be in production already 2023. NAWA Technologies 2022 http://www.nawatechnologies.com/wp-content/uploads/2019/10/NAWA-2019-brochure-Final-092019.pdf
Silicon Anode Batteries
Researchers at the University of Eastern Finland have developed a new hybrid material of mesoporous silicon (PSi) microparticles and carbon nanotubes (CNTs) that can improve the performance of silicon in Li-ion batteries. Silicon will gradually replace carbon as an anode material, because it has ten times larger capacity than graphite but silicon has unstable material properties and production technology is not available to produce anodes solely from silicon. The new hybrid material is realized through chemical conjugation of PSi and CNT with the right polarity so as not to hinder the diffusion of lithium ions into silicon. With the right type of conjugation also the electrical conductivity and mechanical durability of the material were improved. University of Eastern Finland 2022 https://www.uef.fi/en/article/new-hybrid-material-improves-the-performance-of-silicon-in-li-ion-batteries
Zinc-air Batteries
Sydney University School of Chemistry spin-out Gelion is planning to manufacture and distribute the Gelion zinc-bromide battery for Australian market. The zinc-bromide chemistry is reimagined and flowing electrolyte is replaced with a stable gel. This makes the battery far more inexpensive, safe, robust and recyclable in stationary applications free of cooling and maintenance systems. Sydney University 2022 https://www.sydney.edu.au/news-opinion/news/2021/09/10/university-spin-out-gelion-zinc-bromide-batteries-battery-energy-sydney-renewables.html
Other Power Sources of EV:s
Different car manufacturers have established different approaches to determine their strategies of vehicle driving force in green transition. Japanese manufacturers have been leading developers of hybrid and fuel cell technologies but European manufacturers have followed Tesla´s example to concentrate solely on battery EVs. A probable reason for this is the European Union´s challenging goal to end manufacturing of combustion engine powered vehicles by 2035. Automotive News Europe https://europe.autonews.com/environmentemissions/eu-countries-uphold-co2-plan-phases-out-combustion-cars-2035?utm_source=breaking-news&utm_medium=email&utm_campaign=20220629&utm_content=hero-readmore
Dr. Gill Pratt, Chief scientist of Toyota Motor Co. and CEO of Toyota Research Institute provided practical insights to Toyota´s global approach to decarbonization during Toyota Kenshiki Forunm 2022: multiple electrified technologies maximize carbon reduction and simultaneously optimize the utilization of scarce mineral resources in materials needed. Current short supply and high costs of battery materials and the lack of infrastructure are best exploited in a combination of hybrid, plug-in-hybrid and battery electric vehicles and fuel cell vehicles to reduce CO2 emissions over the next 10-15 years, because the “carbon is the enemy, not any particular powertrain”. https://newsroom.toyota.eu/toyota-motor-europe-on-track-to-achieve-carbon-neutrality-by-2040/
Gerald Killman, R&D Director of Toyota Motor Europe was also interviewd and he stated that Mirai Fuel Cell is already tested in several Mirai-Taxis in Japan and mileage shows that fuel cells are made to last for the lifetime of a vehicle (typically 250000-300.000 km), but also use and type of the vehicle matters; for example heavy duty vehicles have different demands. The operation range is comparable to BEV´s but hydrogen fuel refilling time compares better with fossil fuel vehicles than BEV´s. Only the cold climate operation still faces some challenges.
Ni-MH batteries are also used in the future and new bipolar technology is already in use in Japan. Bi-polar technology uses an electrode that is both cathode and anode at the same time and can be used in lead acid and li-ion chemistries while the size is more compact. Li-ion batteries will be used in all different types of EV´s and tendency is that the energy density is growing.
Solid state batteries are the next step forward: there is no liquid electrolyte which restricts electron movement. Therefore they are more efficient and but also safer and easier to recycle, but there is no tested solution yet. Biggest challenge is the discharge/recharge process that should be more reversible and causes short lifespan of battery for the time being. Suomen autolehti 2023
References
Battery university https://batteryuniversity.com/
Chalmers University 2022 https://www.chalmers.se/en/departments/ims/news/Pages/Big-breakthrough-for-%E2%80%99massless%E2%80%99-energy-storage.aspx
Epec Engineered Technologies 2022 https://www.epectec.com/batteries/cell-comparison.html
Green Cars https://www.greencars.com/greencars-10/the-future-of-ev-batteries
Insideevs.com https://insideevs.com/news/598656/tesla-4680-battery-cell-specs/
H.Duong, J.Shin & Y.Yud 2018 Dry Electrode Coating Technology http://www.powersourcesconference.com/Power%20Sources%202018%20Digest/docs/3-1.pdf
Munro & Associates 2022 https://www.youtube.com/watch?v=VEBY7rEUZiI
NAWA Technologies 2022 http://www.nawatechnologies.com/wp-content/uploads/2019/10/NAWA-2019-brochure-Final-092019.pdf
Suomen autolehti 1/2023: Interview of Gerald Killmann p.18-19
Sydney University 2022 https://www.sydney.edu.au/news-opinion/news/2021/09/10/university-spin-out-gelion-zinc-bromide-batteries-battery-energy-sydney-renewables.html
University of Eastern Finland 2022 https://www.uef.fi/en/article/new-hybrid-material-improves-the-performance-of-silicon-in-li-ion-batteries
Conclusion
Smart Charge project has been a very interesting exercise on joint Nordic collaboration with global pandemic as a sideshow. Project management with national travelling restrictions over the borders has dramatically prevented planned face-to-face gatherings but remote tele-meetings have enabled the project to proceed and planned actions to happen in a slightly different way than originally imagined.
Vehicle to Grid (V2G) chargers have been acquired, standards of V2G communication have been studied and data exchange protocol softwares updated until operation and performance have been at an approvable level. Setups have been tested and reassembled in the pilot test site. During normal operation of e-sled snowmobile safaris the charging data has been collected and routed to Lapland UAS cloud platform and saved to database. Data visualization has been developed to illustrate the flow of electricity from source to another and backwards when necessary.
Arctic University of Norway (UiT), Narvik campus, has studied collected data from two pilot sites and evaluated the results. They have prepared several scientific papers about measured data and conclusions about V2G viability in Arctic circumstances.
A lot of information has been also distributed in several webinars about the project and the results discovered. Multiple companies in the sector have been involved in the project with the steering group actions and especially with practical work when pilot sites have been developed, tested and operated.
Although V2G and similar solutions (V2X) have been on the market for at least five years not so many success stories have been reported yet. “The business and revenue model has not been discovered yet” said a representative of exhibitors in the fair of EVs and batteries.
But the time will come.